I’ve been fascinated by glaciers since I was 14, when geography textbooks taught me about strange rivers of ice that crept down yawning valleys like giant serpents stalking their next meal. That kernel of wonder has carried me through a career of more than 25 years. I’ve travelled to the world’s peaks and its poles to see over 20 glaciers. Yet, when I first started out as a researcher in the early 1990s, we were convinced glaciers were lifeless deserts.
Then in 1999, Professor Martin Sharp and colleagues discovered bacteria living beneath the Haut Glacier d’Arolla in Switzerland. It seemed that glaciers, like the soil or our stomachs, had their own community of microbes, their own microbiome. Since then, we’ve found microorganisms just about everywhere within glaciers, transforming what we thought were sterile wastelands into vibrant ecosystems.
So what’s all that glacier life doing? These life forms may be invisible to the naked eye, but they can control how fast glaciers melt – and may even influence the global climate.
The glacier microbiome
Just like people, glacier microbes modify their homes. When I first saw the melting fringes of Greenland’s vast ice sheet, it looked as if a dust storm had scattered a vast blanket of dirt on the ice. Our team later discovered the dirt included extensive mats of glacier algae. These microscopic plant-like organisms contain pigments to help them harvest the Sun’s rays and protect them from harsh UV radiation. By coating the melting ice surface, they darken it, ensuring the ice absorbs more sunlight which causes more of it to melt. In western Greenland, more than 10% of the summer ice melt is caused by algae.
Grzegorz Lis, Author provided
Again, just like us, microbes extract things from their environment to survive. The murky depths of glaciers are among the most challenging habitats for life on Earth. Microbes called chemolithotrophs – from the Greek meaning “eaters of rock” – survive here without light and get their energy from breaking down rock, releasing vital nutrients like iron, phosphorous and silicon to the meltwater.
Rivers and icebergs carry these nutrients to the ocean where they sustain the plant-like phytoplankton – the base of marine food webs which ultimately feed entire ecosystems, from microscopic animals, to fish and even whales. Models and satellite observations show a lot of the photosynthesis in the iron-starved Southern Ocean could be sustained by rusty icebergs and meltwaters, which contain iron unlocked by glacier microbes. Recent evidence suggests something similar occurs off west and east Greenland too.
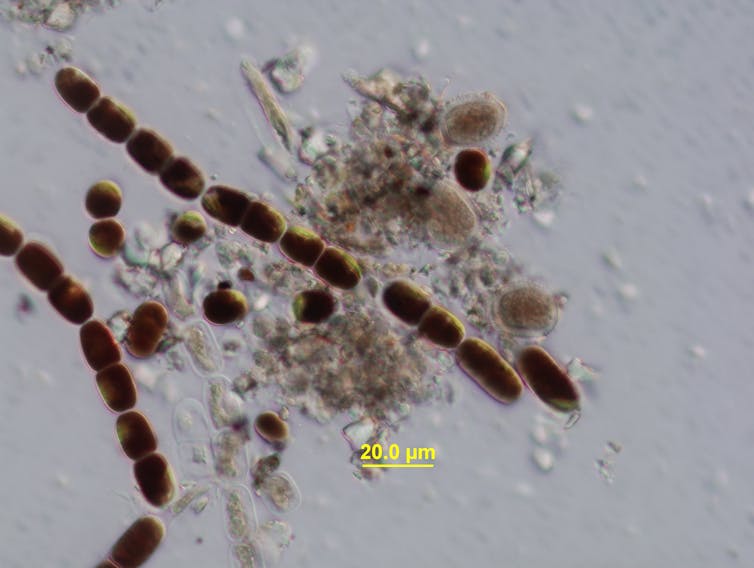
Chris Williamson, Author provided
But glacier bugs also produce waste, the most worrying of which is the greenhouse gas methane. When ice sheets grow, they bury old soils and sediments, all sources of carbon and the building blocks for earthly life. We think there could be thousands of billions of tonnes of carbon buried beneath ice sheets – potentially more than Arctic permafrost. But who can use it in the oxygen-starved belly of an ice sheet? One type of microbe that flourishes here is the methanogen (meaning “methane maker”), which also thrives in landfill sites and rice paddies.
Jemma Wadham, Author provided
Some methane produced by methanogens escapes in meltwaters flowing from the ice sheet edges. The clever thing about microbial communities, though, is that one microbe’s waste is another’s food. We humans could learn a lot from them about recycling. Some methane beneath glaciers is consumed by bacteria called methanotrophs (methane eaters) which generate energy by converting it to carbon dioxide. They have been detected in Greenlandic glaciers, but most notably in Lake Whillans beneath the West Antarctic Ice Sheet. Here, bacteria have years to chomp on the gas, and almost all of the methane produced in the lake is eaten – a good thing for the climate, since carbon dioxide is 80 times less potent as a greenhouse gas when measured over two decades.
We’re not sure this happens everywhere though. Fast-flowing rivers emerging from the Greenland Ice Sheet are super-saturated with microbial methane because there just isn’t enough time for the methanotrophs to get to work. Will melting glaciers release stored methane faster than these bacteria can convert it?
Within the thick interior of ice sheets, scientists worry that there may be vast reserves of methane. The cold and high pressure here mean that it may be trapped in its solid form, methane hydrate (or clathrate), which is stable unless the ice retreats and thins. It happened before and it could happen again.
Waking the sleeping giant
Despite the climate crisis, when I spend time around glaciers I’m not surprised by their continuing vitality. As I amble up to the gently sloping snout of a glacier – traversing its rubbly lunar-like fore-fields – I often feel like I’m approaching the hulk of an enormous creature. Sleeping or seemingly dormant, the evidence of its last meal is clear from the mass of tawny-coloured rocks, pebbles and boulders strewn around its edges – a tantalising record of where it once rested when the climate was cooler.
As I get closer, I catch the sound of the glacier’s roaring chocolate meltwaters as they explode through an ice cave, punctuated by a cascade of bangs and booms as moving ice collapses into hollow melt channels below. The winds off the ice play ominously in my ears, like the whisper of the beast, a warning: “You’re on my land now.”
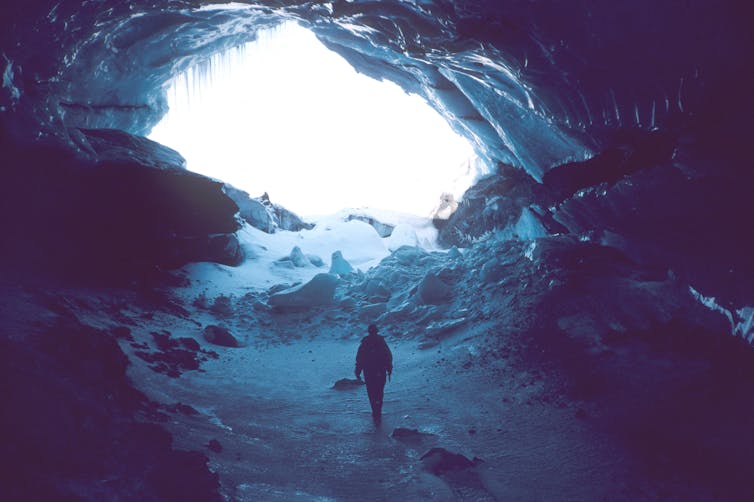
Jon Ove Hagen, Author provided
This sense of aliveness with glaciers changes everything. Resident microbes connect these hulking frozen masses with the Earth’s carbon cycle, ecosystems and climate. How will these connections change if we take away the frigid homes of our tiny glacier dwellers? These creatures may be microscopic, but the effects of their industry span entire continents and oceans.
After a period of uncertainty in my own life, which involved the removal of a satsuma-sized growth in my brain, I felt compelled to tell the story of glaciers to a wider audience. My book, Ice Rivers, is the result. I hope the memoir raises awareness of the dramatic changes that threaten glaciers – unless we act now.
———————————-
This blog is written by Cabot Institute for the Environment Director Jemma Wadham, Professor of Glaciology, University of Bristol.
This article is republished from The Conversation under a Creative Commons license. Read the original article.
![]() |
Professor Jemma Wadham |