Frank Herbert’s Dune is epic sci-fi storytelling with an environmental message at its heart. The novels and movies are set on the desert planet of Arrakis, which various characters dream of transforming into a greener world – much like some envision for Mars today.
We investigated Arrakis using a climate model, a computer program similar to those used to give weather forecasts. We found the world that Herbert had created, well before climate models even existed, was remarkably accurate – and would be habitable, if not hospitable.
However, Arrakis wasn’t always a desert. In Dune lore, 91% of the planet was once covered by oceans, until some ancient catastrophe led to its desertification. What water remained was further removed by sand trout, an invasive species brought to Arrakis. These proliferated and carried liquid into cavities deep underground, leading to the planet becoming more and more arid.
To see what a large ocean would mean for the planet’s climate and habitability, we have now used the same climate model – putting in an ocean while changing no other factors.
When most of Arrakis is flooded, we calculate that the global average temperature would be reduced by 4°C. This is mostly because oceans add moisture to the atmosphere, which leads to more snow and certain types of cloud, both of which reflect the sun’s energy back into space. But it’s also because oceans on Earth and (we assume) on Arrakis emit “halogens” that cool the planet by depleting ozone, a potent greenhouse gas which Arrakis would have significantly more of than Earth.
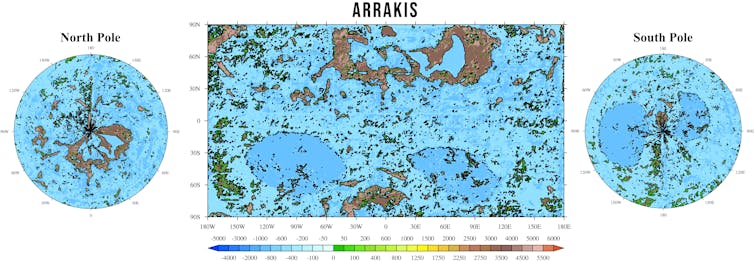
Farnsworth et al, CC BY-SA
Unsurprisingly, the ocean world is a whopping 86 times wetter, as so much water evaporates from the oceans. This means plants can grow as water is no longer a finite resource, as it is on desert Arrakis.
A wetter world would be more stable
Oceans also reduce temperature extremes, as water heats and cools more slowly than land. (This is one reason Britain, surrounded by oceans, has relatively mild winters and summers, while places far inland tend to be hotter in summer and very cold in winter). The climate of an ocean planet is therefore more stable than a desert world.
In desert Arrakis, temperatures would reach 70°C or more, while in its ocean state, we put the highest recorded temperatures at about 45°C. That means the ocean Arrakis would be liveable even in summer. Forests and arable crops could grow outside of the (still cold and snowy) poles.
There is one downside, however. Tropical regions would be buffeted by large cyclones since the huge, warm oceans would contain lots of the energy and moisture required to drive hurricanes.
The search for habitable planets
All this isn’t an entirely abstract exercise, as scientists searching for habitable “exoplanets” in distant galaxies are looking for these sorts of things too. At the moment, we can only detect such planets using huge telescopes in space to search for those that are similar to Earth in size, temperature, available energy, ability to host water, and other factors.
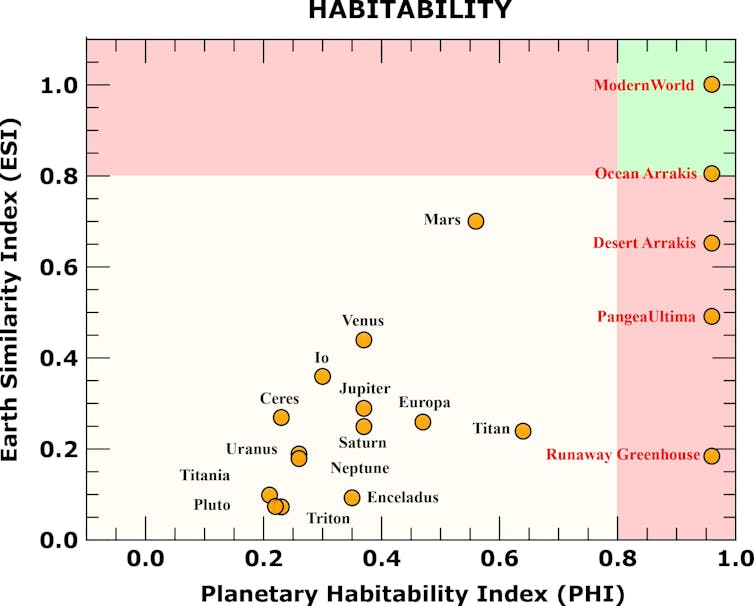
Farnsworth et al, CC BY-SA
We know that desert worlds are probably more common than Earth-like planets in the universe. Planets with potentially life-sustaining oceans will usually be found in the so-called “Goldilocks zone”: far enough from the Sun to avoid being too hot (so further away than boiling hot Venus), but close enough to avoid everything being frozen (so nearer than Jupiter’s icy moon Ganymede).
Research has found this habitable zone is particularly small for planets with large oceans. Their water is at risk of either completely freezing, therefore making the planet even colder, or of evaporating as part of a runaway greenhouse effect in which a layer of water vapour prevents heat from escaping and the planet gets hotter and hotter.
The habitable zone is therefore much larger for desert planets, since at the outer edge they will have less snow and ice cover and will absorb more of their sun’s heat, while at the inner edge there is less water vapour and so less risk of a runaway greenhouse effect.
It’s also important to note that, though distance from their local star can give a general average temperature for a planet, such an average can be misleading. For instance, both desert and ocean Arrakis have a habitable average temperature, but the day-to-day temperature extremes on the ocean planet are much more hospitable.
Currently, even the most powerful telescopes cannot sense temperatures at this detail. They also cannot see in detail how the continents are arranged on distant planets. This again could mean the averages are misleading. For instance, while the ocean Arrakis we modelled would be very habitable, most of the land is in the polar regions which are under snow year-round – so the actual amount of inhabitable land is much less.
Such considerations could be important in our own far-future, when the Earth is projected to form a supercontinent centred on the equator. That continent would make the planet far too hot for mammals and other life to survive, potentially leading to mass extinction.
If the most likely liveable planets in the universe are deserts, they may well be very extreme environments that require significant technological solutions and resources to enable life – desert worlds will probably not have an oxygen-rich atmosphere, for instance.
But that won’t stop humans from trying. For instance, Elon Musk and SpaceX have grand ambitions to create a colony on our closest desert world, Mars. But the many challenges they will face only emphasises how important our own Earth is as the cradle of civilisation – especially as ocean-rich worlds may not be as plentiful as we’d hope. If humans eventually colonise other worlds, they’re likely to have to deal with many of the same problems as the characters in Dune.
————————
This blog is written by Cabot Institute for the Environment members Dr Alex Farnsworth, Senior Research Associate in Meteorology, and Sebastian Steinig, Research Associate in Paleoclimate Modelling, University of Bristol; and Michael Farnsworth, Research Lead Future Electrical Machines Manufacturing Hub, University of Sheffield. This article is republished from The Conversation under a Creative Commons license. Read the original article.