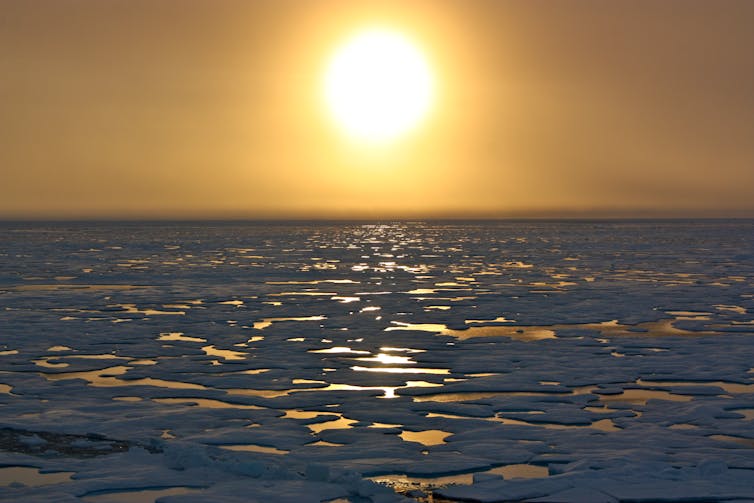
NASA Goddard Space Flight Center
The Arctic Ocean could be ice-free in summer by the 2030s, even if we do a good job of reducing emissions between now and then. That’s the worrying conclusion of a new study in Nature Communications.
Predictions of an ice-free Arctic Ocean have a long and complicated history, and the 2030s is sooner than most scientists had thought possible (though it is later than some had wrongly forecast). What we know for sure is the disappearance of sea ice at the top of the world would not only be an emblematic sign of climate breakdown, but it would have global, damaging and dangerous consequences.
The Arctic has been experiencing climate heating faster than any other part of the planet. As it is at the frontline of climate change, the eyes of many scientists and local indigenous people have been on the sea ice that covers much of the Arctic Ocean in winter. This thin film of frozen seawater expands and contracts with the seasons, reaching a minimum area in September each year.
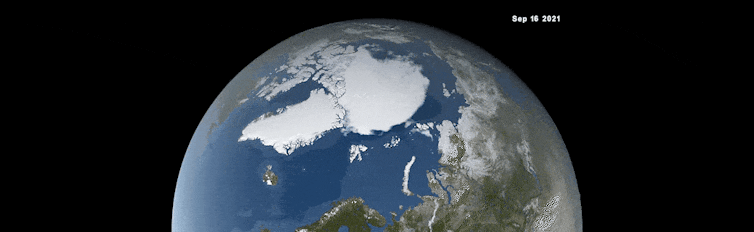
NASA
The ice which remains at the end of summer is called multiyear sea ice and is considerably thicker than its seasonal counterpart. It acts as barrier to the transfer of both moisture and heat between the ocean and atmosphere. Over the past 40 years this multiyear sea ice has shrunk from around 7 million sq km to 4 million. That is a loss equivalent to roughly the size of India or 12 UKs. In other words, it’s a big signal, one of the most stark and dramatic signs of fundamental change to the climate system anywhere in the world.
As a consequence, there has been considerable effort invested in determining when the Arctic Ocean might first become ice-free in summer, sometimes called a “blue ocean event” and defined as when the sea ice area drops below 1 million sq kms. This threshold is used mainly because older, thicker ice along parts of Canada and northern Greenland is expected to remain long after the rest of the Arctic Ocean is ice-free. We can’t put an exact date on the last blue ocean event, but one in the near future would likely mean open water at the North Pole for the first time in thousands of years.
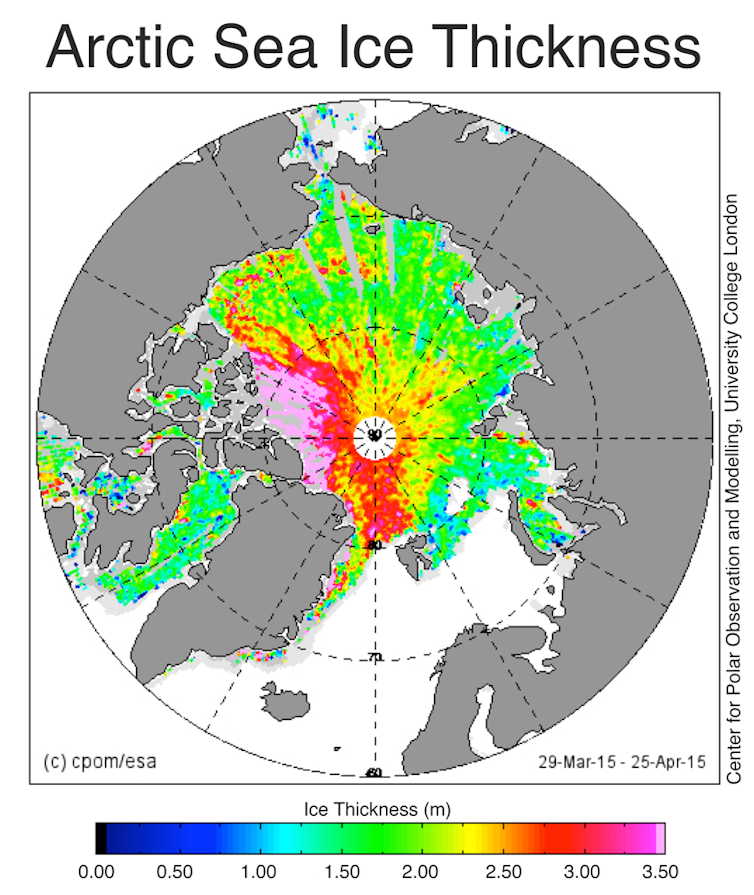
NERC Center for Polar Observation and Modelling, CC BY-SA
One problem with predicting when this might occur is that sea ice is notoriously difficult to model because it is influenced by both atmospheric and oceanic circulation as well as the flow of heat between these two parts of the climate system. That means that the climate models – powerful computer programs used to simulate the environment – need to get all of these components right to be able to accurately predict changes in sea ice extent.
Melting faster than models predicted
Back in the 2000s, an assessment of early generations of climate models found they generally underpredicted the loss of sea ice when compared to satellite data showing what actually happened. The models predicted a loss of about 2.5% per decade, while the observations were closer to 8%.
The next generation of models did better but were still not matching observations which, at that time were suggesting a blue ocean event would happen by mid-century. Indeed, the latest IPCC climate science report, published in 2021, reaches a similar conclusion about the timing of an ice-free Arctic Ocean.
As a consequence of the problems with the climate models, some scientists have attempted to extrapolate the observational record resulting in the controversial and, ultimately, incorrect assertion that this would happen during the mid 2010s. This did not help the credibility of the scientific community and its ability to make reliable projections.
Ice-free by 2030?
The scientists behind the latest study have taken a different approach by, in effect, calibrating the models with the observations and then using this calibrated solution to project sea ice decline. This makes a lot of sense, because it reduces the effect of small biases in the climate models that can in turn bias the sea ice projections. They call these “observationally constrained” projections and find that the Arctic could become ice-free in summer as early as 2030, even if we do a good job of reducing emissions between now and then.
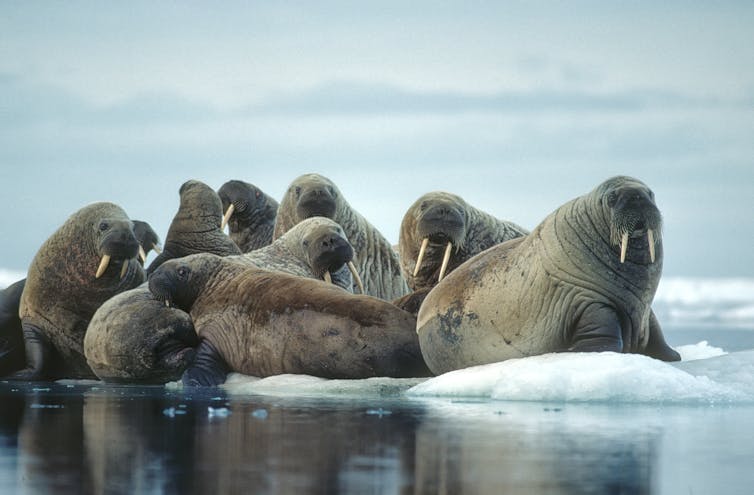
outdoorsman / shutterstock
There is still plenty of uncertainty around the exact date – about 20 years or so – because of natural chaotic fluctuations in the climate system. But compared to previous research, the new study still brings forward the most likely timing of a blue ocean event by about a decade.
Why this matters
You might be asking the question: so what? Other than some polar bears not being able to hunt in the same way, why does it matter? Perhaps there are even benefits as the previous US secretary of state, Mike Pompeo, once declared – it means ships from Asia can potentially save around 3,000 miles of journey to European ports in summer at least.
But Arctic sea ice is an important component of the climate system. As it dramatically reduces the amount of sunlight absorbed by the ocean, removing this ice is predicted to further accelerate warming, through a process known as a positive feedback. This, in turn, will make the Greenland ice sheet melt faster, which is already a major contributor to sea level rise.
The loss of sea ice in summer would also mean changes in atmospheric circulation and storm tracks, and fundamental shifts in ocean biological activity. These are just some of the highly undesirable consequences and it is fair to say that the disadvantages will far outweigh the slender benefits.
This blog is written by Cabot Institute for the Environment member Jonathan Bamber, Professor of Physical Geography, University of Bristol. This article is republished from The Conversation under a Creative Commons license. Read the original article.
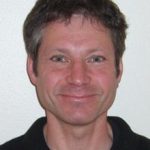