Animals can only endure temperatures within a given range. The upper and lower temperatures of this range are called its critical thermal limits. As these limits are exceeded, an animal must either adjust or migrate to a cooler climate.
However, temperatures are rising across the world at a rapid pace. The record-breaking heatwaves experienced across Europe this summer are indicative of this. Heatwaves such as these can cause temperatures to regularly surpass critical thermal limits, endangering many species.
In a new study, my colleagues and I assessed how well 102 species of insect can adjust their critical thermal limits to survive temperature extremes. We found that insects have a weak capacity to do so, making them particularly vulnerable to climate change.
The impact of climate change on insects could have profound consequences for human life. Many insect species serve important ecological functions while the movement of others can disrupt the balance of ecosystems.
How do animals adjust to temperature extremes?
An animal can extend its critical thermal limits through either acclimation or adaptation.
Acclimation occurs within an animal’s lifetime (often within hours). It’s the process by which previous exposure helps give an animal or insect protection against later environmental stress. Humans acclimate to intense UV exposure through gradual tanning which later protects skin against harmful UV rays.
One way insects acclimate is by producing heat shock proteins in response to heat exposure. This prevents cells dying under temperature extremes.
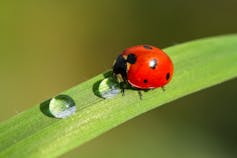
mehmetkrc/Shutterstock
Some insects can also use colour to acclimate. Ladybirds that develop in warm environments emerge from the pupal stage with less spots than insects that develop in the cold. As darker spots absorb heat, having fewer spots keeps the insect cooler.
Adaptation occurs when useful genes are passed through generations via evolution. There are multiple examples of animals evolving in response to climate change.
Over the past 150 years, some Australian parrot species such as gang-gang cockatoos and red-rumped parrots have evolved larger beaks. As a greater quantity of blood can be diverted to a larger beak, more heat can be lost into the surrounding environment.
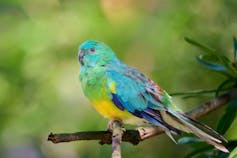
Alamin-Khan/Shutterstock
But evolution occurs over a longer period than acclimation and may not allow critical thermal limits to adjust in line with the current pace of global temperature rise. Upper thermal limits are particularly slow to evolve, which may be due to the large genetic changes required for greater heat tolerance.
Research into how acclimation might help animals survive exceptional temperature rise has therefore become an area of growing scientific interest.
A weak ability to adjust to temperature extremes
When exposed to a 1℃ change in temperature, we found that insects could only modify their upper thermal limit by around 10% and their lower limit by around 15% on average. In comparison, a separate study found that fish and crustaceans could modify their limits by around 30%.
But we found that there are windows during development where an insect has a greater tolerance towards heat. As juvenile insects are less mobile than adults, they are less able to use their behaviour to modify their temperature. A caterpillar in its cocoon stage, for example, cannot move into the shade to escape the heat.
Exposed to greater temperature variations, this immobile life stage has faced strong evolutionary pressure to develop mechanisms to withstand temperature stress. Juvenile insects generally had a greater capacity for acclimating to rising temperatures than adult insects. Juveniles were able to modify their upper thermal limit by 11% on average, compared to 7% for adults.
But given that their capacity to acclimate is still relatively weak and may fall as an insect leaves this life stage, the impact is likely to be limited for adjusting to future climate change.
What does this mean for the future?
A weak ability to adjust to higher temperatures will mean many insects will need to migrate to cooler climates in order to survive. The movement of insects into new environments could upset the delicate balance of ecosystems.
Insect pests account for the loss of 40% of global crop production. As their geographical distribution changes, pests could further threaten food security. A UN report from 2021 concluded that fall armyworm populations, which feed on crops such as maize, have already expanded their range due to climate change.
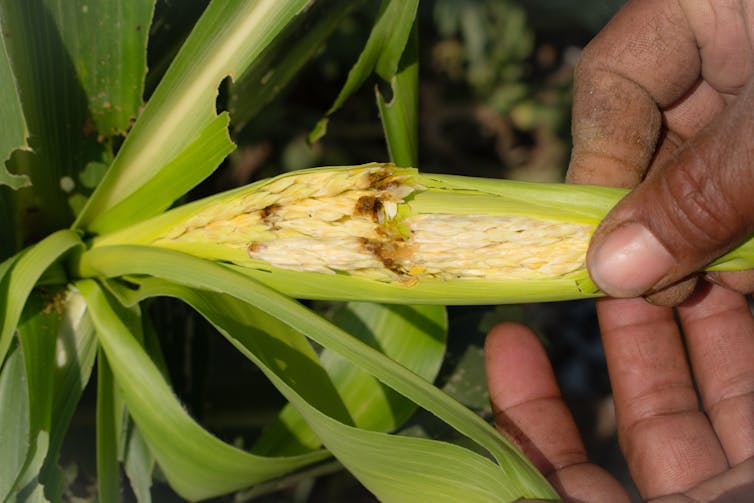
Alchemist from India/Shutterstock
Insect migration may also carry profound impacts on human health. Many of the major diseases affecting humans, including malaria, are transmitted by insects. The movement of insects over time increases the possibility of introducing infectious diseases to higher latitudes.
There have been over 770 cases of West Nile virus recorded in Europe this year. Italy’s Veneto region, where the majority of the cases originate, has emerged as an ideal habitat for Culex mosquitoes, which can host and transmit the virus. Earlier this year, scientists found that the number of mosquitoes in the region had increased by 27%.
Insect species incapable of migrating may also become extinct. This is of concern because many insects perform important ecological functions. Three quarters of the crops produced globally are fertilised by pollinators. Their loss could cause a sharp reduction in global food production.
The vulnerability of insects to temperature extremes means that we face an uncertain and worrying future if we cannot curb the pace of climate change. A clear way of protecting these species is to slow the pace of climate change by reducing fossil fuel consumption. On a smaller scale, the creation of shady habitats, which contain cooler microclimates, could provide essential respite for insects facing rising temperatures.
—————————-
This blog is written by Hester Weaving, PhD Candidate in Entomology, University of Bristol. This article is republished from The Conversation under a Creative Commons license. Read the original article.
![]() |
Hester Weaving |